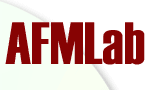
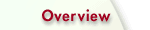
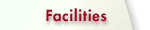
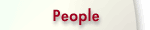
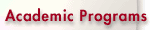
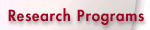
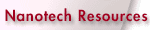
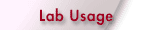
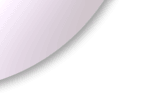
|
 |
 |

Research Programs
-
Response and evolution of molecules and
microstructures
We have investigated how molecules and microstructures -- from the
vital to the virulent --
respond to excitation: hemoglobin to carbon dioxide and
oxygen, carbon
nanotubes to shear, bacteriorhodopsin to light, liquid crystals and
bacterial exopolymers
to strain, microstructures in asphalt binders to temperature and time,
and grains within perovskite solar cells to pressure.
[Ref. 42, 43, 46, 51, 52,
53, 58, 61, 63 in
journal list.]
-
Adhesive and
visco-elastic properties
of nanomaterials
Not
only the chemical environment, but also the topographic and mechanical
environments
of cells influence their growth into tissues. We
have characterized the mechanical properties of polyacrylimide gels on
which cells are grown.
Similarly, the adhesive asphalt binder that holds roads together is
visco-elastic, and
we have monitored the evolution of its microstructures -- no wonder
there are so many potholes in roads! Source
rocks for oil and gas production are likewise nanomaterials with
intriguing properties, which, if better known, could improve the
efficiency and safety with which these non-renewables can be extracted.
[Refs.
39, 50, 54, 56, 59, 60, 62, 64 in journal list.]
-
Topography and device performance
Silicon-based
microsensors can be fabricated with moving devices that
can make contact with the substrate upon which they are grown. Their
surfaces
are coated with a molecular layer that lowers the likelihood
of
sticking and thus prevents failure of the device. We investigated
the contribution
of surface
roughness to the "stiction" using the tip of
an AFM to replace one side of the silicon-oxide interface.
Likewise,
topography changes the capacitance of self-assembled monolayer devices.
[Refs. 38, 40, 41, 45 in journal and Refs. 12, 13 in proceedings list.]
-
Instrumentation and metrology for nanomechanics
Early versions of atomic-force microscopes (AFMs) measured forces
precisely, yet without good accuracy. We developed a
method that quickly calibrates the normal spring constant of the
cantilevers used to
measure forces in AFM to an accuracy of 10%. We also developed a method
to quantitatively measure lateral forces. Other progress lies in
tip-radius calibration, the use of optical excitation at the tip-sample
interface, and the influence of higher modes of the cantilever on its
response. Here are links for programs for tip-radius and
lateral-force calibration.
[Refs. 32-37, 40, 44, 47, 48 in journal list.]
-
Physics and nanoscience education
Introductory physics is challenging to teach, particularly when there
are large numbers of students. Concerning nanoscience, the
creation of
in-person and online content for AFM education has been a labor of love.
[Refs. 49,
55, 57 in
journal, Ref. 8 in book, Ref. 14 in proceedings, and Refs.43, 46 in
project list. YouTube AFM
lectures.]
-
Nanoscience and
society
There
are technologies that have not universally accepted by the public, for
example, vaccines, nuclear power, and genetically modified foods. The
public is currently generally content with
nanoscience, although people balk at the thought of injecting
"nanobots" into their bodies. The level of precautions taken in
nanoscience
research labs has been inconsistent thus far.
[Refs. 11, 13, 19, 22,
26, 41, and 42 in project list.]
![[WPI]](seal.gif) ![[Back]](Buttons/back.gif)
nab@wpi.edu
Last modified: July 2020
|
 |